Improvement of specific capacity of lithium iron phosphate battery by increasing the surface area and electrical conductivity of cathode electrode using graphene foam
DOI:
https://doi.org/10.55713/jmmm.v33i4.1779Keywords:
graphene foam, lithium iron phosphate, lithium-ion battery, chemical vapor depositionAbstract
Lithium iron phosphate (LFP) is widely used as an active material in a cathode electrode for lithium-ion batteries (LIBs). LFP has many remarkable properties such as high working voltage and excellent thermal stability. However, it suffers with slow ion diffusion and low electrical conductivity. Graphene foam has many outstanding properties such as large surface area and great electrical conductivity. These properties are suitable for improving the cathode electrode. In this work, the graphene foam was synthesized by chemical vapor deposition. The cathode electrode was prepared by dropping the LFP on the graphene foam. We found that the specific capacity of battery which contained the LFP between the anode and the graphene foam (LFP/GF) was 23.1 mAh⸳g-1 at 3C, while the specific capacity of battery which contained the graphene foam between the anode and the LFP (GF/LFP) was 112.6 mAh⸳g-1 at 3C. The diffusion coefficients of Li+ of GF/LFP was 9.1 times higher than that of LFP/GF. The specific capacity of GF/LFP was higher than that of LFP/GF at high current density due to the high ion transfer rate which arises from the graphene foam.
Downloads
References
B. Scrosati, and J. Garche, “Lithium batteries: Status, prospects and future,” Journal of Power Sources, vol. 195, pp. 2419-2430, 2010.
A. K. Padhi, K. S. Nanjundaswamy, and J. B. Goodenough, “Phospho-olivines as Positive-Electrode materials for rechargeable Lithium batteries,” Journal of The Electrochemical Society, vol. 144, pp. 1188-1194, 1997.
J. M. Tarascon, and M. Armand, “Issues and challenges facing rechargeable lithium batteries,” Nature, vol. 414, pp. 359-367, 2001.
H. Raj, and A. Sil, “Effect of carbon coating on electrochemical performance of LiFePO4 cathode material for Li-ion battery,” Ionics, vol. 24, no. 26, pp. 2543-2553, 2018.
J. F. Ni, H. H. Zhou, J. T. Chen, and X. X. Zhang, “LiFePO4 doped with ions prepared by co-precipitation method,” Materials Letters, vol. 59, pp. 2361-2365, 2005.
Z. Tian, S. Liu, F. Ye, S. Yao, Z. Zhou, and S. Wang, “Synthesis and characterization of LiFePO4 electrode materials coated by graphene,” Applied Surface Science, vol. 305, pp. 427-432, 2014.
H. Ji, L. Zhang, M. T. Pettes, H. Li, S. Chen, L. Shi, R. Piner, and R. S. Ruoff, “Ultrathin graphite foam: A Three-dimensional conductive network for battery electrodes,” Nano Letters, vol. 12, pp. 2446-2451, 2012.
A. K. Geim, and K. S. Novoselov, “The rise of graphene,” Nature Materials, vol. 6, pp. 183-191, 2007.
A. A. Balandin, S. Ghosh, W. Bao, I. Calizo, D. Teweldebrhan, F. Miao, and C. N. Lau, “Superior thermal conductivity of single-layer graphene,” Nano Letters, vol. 8, pp. 902-907, 2008.
T. Ohta, A. Bostwick, J. L. McChesney, T. Seyller, K. Horn, and E. Rotenberg, “Interlayer interaction and electronic screening in multilayer graphene investigated with angle-resolved photo-emission spectroscopy,” Physical Review Letters, vol. 98, pp. 206802, 2007.
A. Ruammaitree, H. Nakahara, K. Akimoto, K. Soda, and Y. Saito, “Determination of non-uniform graphene thickness on SiC (0001) by X-ray diffraction,” Applied Surface Science, vol. 282, pp. 297-301, 2013.
K. V. Emtsev, F. Speck, Th. Seyller, and L. Ley, “Interaction, growth, and ordering of epitaxial graphene on SiC{0001} surfaces: A comparative photoelectron spectroscopy study,” Physical Review B, vol. 77, p. 155303, 2008.
L. Zhou, Z. Yang, J. Yang, Y. Wu, and D. Wei, “Facile syntheses of 3-dimension graphene aerogel and nanowalls with high specific surface areas,” Chemical Physics Letters, vol. 677, pp. 7-12, 2017.
I. A. Ovid’ko, “Mechanical properties of graphene,” Reviews on Advanced Materials Science, vol. 34, 1-11, 2013.
A. Ruammaitree, H. Nakahara, and Y. Saito, “Growth of protrusive graphene rings on Siterminated 6H–SiC (0001),” Surface and Interface Analysis, vol. 46, pp. 1156-1159, 2014.
A. Ruammaitree, H. Nakahara, and Y. Saito, “Growth of non-concentric graphene ring on 6H-SiC (0001) surface,” Applied Surface Science, vol. 307, pp. 136-141, 2014.
H. Hu, A. Ruammaitree, H. Nakahara, K. Asaka, and Y. Saito, “Few-layer epitaxial graphene with large domains on C-terminated 6H-SiC,” Surface and Interface Analysis, vol. 44, pp. 793-796, 2012.
P. Surinlert, P. Kokmatb, and A. Ruammaitree, “Growth of turbostratic stacked graphene using waste ferric chloride solution as a feedstock,” RSC Advances, vol. 12, pp. 25048-25053, 2022.
P. Kokmat, P. Surinlert, and A. Ruammaitree, “Growth of high-purity and high-quality turbostratic graphene with different interlayer spacings,” ACS Omega, vol. 8, pp. 4010-4018, 2023.
A. Ruammaitree, D. Phokharatkul, and A. Wisitsoraat, “Surface hardening of stainless steel by coating graphene using thermal chemical vapor deposition,” Solid State Phenomena, vol 283, pp. 173-178, 2018.
P. Kokmat, N. Donnuea, N. Nuntawong, A. Wisitsoraat, and A. Ruammaitree, “Effect of acetic acid pre-treatment on the growth temperature of graphene on copper by thermal chemical vapor deposition,” IOP Conference Series: Materials Science and Engineering, vol. 526, p. 012008, 2019.
J. Oh, J. Lee, Y. Jeon, S. Park, J. M. Kim, T. Hwang, and Y. Piao, “Melamine foam-derived n‑doped carbon framework and graphene-supported LiFePO4 composite for high performance lithium-ion battery cathode material,” ACS Sustainable Chemistry & Engineering, vol. 7, pp. 306-314, 2019.
M. J. Matthews, and M. A. Pimenta, “Origin of dispersive effects of the Raman D band in carbon materials,” Physical review B, vol. 59, pp. R6585- R6588, 1999.
Y. L. Liu, C. C. Yu, C. Y. Fang, H. L. Chen, C. W. Chen, C. C. Kuo, C. K. Chang, L. C. Chen, and K. H. Chen, “Using optical anisotropy as a quality factor to rapidly characterize structural qualities of large-area graphene films,” Analytical Chemistry, vol. 85, pp. 1605-1614, 2013.
M. A. Pimenta, G. Dresselhaus, M. S. Dresselhaus, L. G. Cancado, A. Jorio, and R. Saito, “Studying disorder in graphite-based systems by Raman spectroscopy,” Physical Chemistry Chemical Physics, vol. 9, pp. 1276-1291, 2007.
C. Cong, T. Yu, R. Saito, G. F. Dresselhaus, and M. S. Dresselhaus, “Second-order overtone and combination raman modes of graphene layers in the range of 1690-2150 cm-1,” ACS Nano, vol. 5, pp. 1600-1605, 2011.
R. Rao, R. Podila, R. Tsuchikawa, J. Katoch, D. Tishler, A. M. Rao, and M. Ishigami, “Effects of layer stacking on the combination Raman modes in Graphene,” ACS nano, vol. 5, pp. 1594-1599, 2011.
B. M. Goh, Y. Wang, M. V. Reddy, Y. L. Ding, L. Lu, C. Bunker, and K. P. Loh, “Filling the voids of graphene foam with graphene “Eggshell” for improved lithium-ion storage,” ACS Applied Materials & Interfaces, vol. 6, pp. 9835-9841, 2014.
J. Lu, X. Tian, Y. Zhou, Y. Zhu, Z. Tang, B. Ma, G. Wu, T. Jiang, X Tu, and G. Z. Chen, “A novel “holey-LFP/graphene/ holey-LFP” sandwich nanostructure with significantly improved rate capability for lithium storage”, Electrochimica Acta, vol. 320, p. 134566, 2019.
G. Luo, Y. Gu, Y. Liu, Z. Chen, Y. Huo, F. Wu, Y. Mai, X. Dai, and Y. Deng, “Electrochemical performance of in situ LiFePO4 modifed by N-doped graphene for Li-ion batteries,” Ceramics International, vol. 47, pp. 11332-11339, 2021.
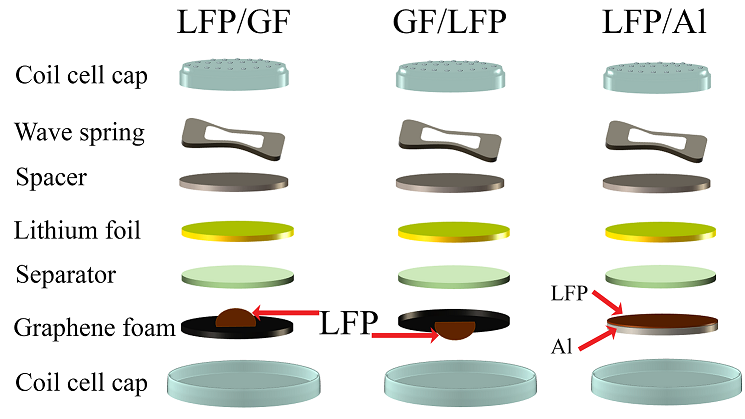
Downloads
Published
How to Cite
Issue
Section
License
Copyright (c) 2023 Journal of Metals, Materials and Minerals

This work is licensed under a Creative Commons Attribution-NonCommercial-NoDerivatives 4.0 International License.
Authors who publish in this journal agree to the following terms:
- Authors retain copyright and grant the journal right of first publication with the work simultaneously licensed under a Creative Commons Attribution License that allows others to share the work with an acknowledgment of the work's authorship and initial publication in this journal.
- Authors are able to enter into separate, additional contractual arrangements for the non-exclusive distribution of the journal's published version of the work (e.g., post it to an institutional repository or publish it in a book), with an acknowledgment of its initial publication in this journal.